by
Esther Mar
| Dec 17, 2018
When producing a part, either by deep drawing, stretch forming or bending, flat sheet is transformed into a design shape and dimension. At the end of the forming process, when the part has been released from the forces of the forming tool, there is a distortion in the shape and dimension of the formed part. This distortion is termed springback. A depiction of springback in a simple bend can be seen in Figure 1.
Springback is inherent in sheet metal forming. It can be can be understood by looking at a material’s stress stain curve (discussed in the module on Tensile Testing) which characterizes the behavior of metal under applied force. During forming, the material is strained beyond the yield strength in order to induce permanent deformation. When the load is removed, the stress will return to zero along a path parallel to the slope of the elastic portion of the curve, which is the elastic modulus. This can be seen in Figure 2. The permanent deformation will therefore be less than what is designed into the part unless springback is factored in.
Springback is dependent on various material characteristics but can be affected by tooling design. The most important parameters are elastic modulus, strength, thickness, and bend radius. Other material characteristics, especially YPE, can also be important.
Material with a higher elastic modulus will show less springback than material with a lower elastic modulus. This can be seen in Figure 2, where the unloading stress strain curve would be shifted toward less springback if it had a higher slope. However between different types of steel there is essentially no difference in the elastic modulus so unless a totally different material is chosen, such as aluminum, this is not a consideration.
A material with a higher yield strength will have a greater ratio of elastic to plastic strain and will exhibit more springback than material with a lower yield strength for a given amount of strain.
Thickness is important because of how it impacts on strain. There is high total strain involved in bending a thick material around a given radius and low total strain involved in bending a thin material around the same radius. While under load, the total strain consists of both elastic and plastic strains. When the total strain is high, based on the stress strain curve, the relative amount of elastic strain is low. When the total strain is low, the relative amount of elastic strain is high and this results in more springback. It can be imagined that a very thin material could be bent around a radius with zero plastic strain. In this case the strain would be so low that it would be entirely elastic and the material would completely spring back to its original shape after the load is removed. Conversely a very thick material being bent over the same radius would show a very high total strain. The absolute value of the elastic portion of the strain would be similar to that of the thin material but it would be insignificant compared to the plastic portion of the strain. Therefore the thick material would show very little springback.
Another material characteristic worth mentioning is the Yield Point Elongation (YPE). YPE is the strain associated with discontinuous yielding that can occur when steel is placed in tension. It is well demonstrated that steel showing a pronounced YPE shows less springback than steel with no YPE. In the case of steel with a high YPE more of the stress is used to concentrate thinning locally resulting in a lower proportion of total strain that is elastic and hence less springback. One would think that this parameter can be used to effectively reduce springback. However that is not always the case because YPE is variable, between coils, within coils, and is directional, so its effects can be variable. In general variability can be more problematic than the absolute value of springback.
Methods of addressing springback include process design and part design. In terms of the process, overbending, retarding metal flow due by use of draw beads and higher binder pressure, using lower press speeds, restriking, applying tension during bending, using tighter die clearances, are all techniques that are used. In terms of part design, by utilizing tooling configurations that force higher strain over a small area springback can be minimized.
As well there are angle compensation feedback mechanisms that are able to make automatic adjustments for each piece.
Finally there are modeling techniques that attempt to analyze three dimensional parts so that the tooling can be designed to compensate for springback.
Figure 1. Elastic Springback (the change in the angle from start to finish of the forming process)
(r = radius, a = angle, s = start, f = finish)
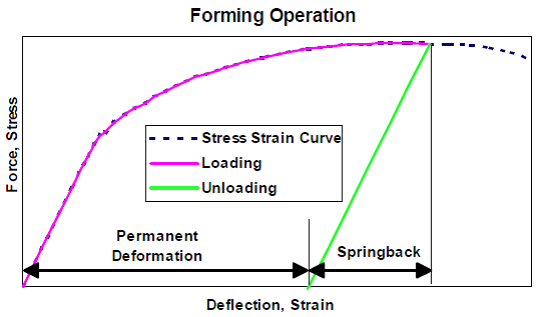
Figure 2. Strain and Stress Behaviour During the Forming Process